Research
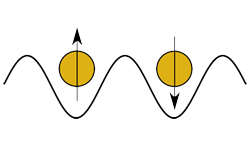
Atomic & Molecular Optics
Study of matter-matter and light-matter interaction on the scale of single atoms or molecules
- Ultra-cold atoms (C-L Hung, D. Elliott, Y. Chen)
- Many-body dynamics (F. Robicheaux, C. Greene)
- Coherent transient effects in atomic ensembles (D. Elliott)
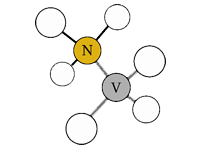
Solid-State Quantum Systems
Study of quantum systems built inside a solid state of matter
- Color centers in diamond (V. Shalaev, T. Li)
- Topological insulators and correlated electron systems (Y. Chen, G.A. Csathy, Y. Lyanda-Geller, L. Rokhinson, R. Biswas)
- Quantum dots (G. Klimeck)
- Quantum optomechanics (T. Li, S. Bhave)
- 2D materials and graphene (Y. Chen, A. Boltasseva, G.A. Csathy)
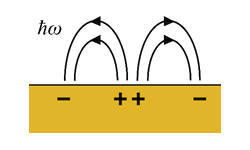
Quantum Nanophotonics
Nanoscale photonic structures for applications in quantum computation, communication, and sensing
- CoE Tellabs Center for Quantum Photonics (TCQP)
- SiN non-linear optics and entangled photon generation (M. Qi, A. Weiner)
- Control of interaction with metamaterials (V. Shalaev, E. Narimanov, A. Kildishev, A. Boltasseva)
- Vacuum Fluctuation Effects (Z. Jacob)
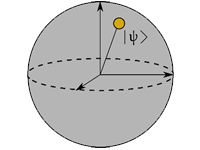
Quantum Information & Communication
Study of quantum mechanical systems which can be used for processing, transmitting, and storing information
- Bi-photon pulse shaping (A. Weiner)
- Quantum algorithms and adiabatic quantum computing (S. Kais, A. Jung)
- Quantum Communications (V. Aggarwal)
People
Leadership
-
Yong Chen - Director of Purdue Quantum Science and Engineering Institute
Karl Lark-Horovitz Professor of Physics and Astronomy, Professor of Electrical and Computer Engineering
BRK 1287B Group Website
The Quantum Matter & Devices (QMD) Lab exploits quantum physics to manipulate electrons, photons and atoms in quantum materials and artificial quantum systems, with the aim to uncover novel quantum phenomena & new states of matter and to explore innovative applications in quantum information processing or nanotechnology (such as nanoelectronics and nanosensors). Our research topics include both nano/solid state physics (graphene & 2D materials, topological insulators), atomic/molecular physics (Bose-Einstein condensates, polar molecules) and related applications.
-
David Stewart
Managing Director of Purdue Quantum Science and Engineering Institute
BRK 1287C
Internal Faculty Advisory Board
-
Christopher Greene - Co-Chair of Internal Faculty Advisory Board
Albert Overhauser Distinguished Professor of Physics and Astronomy
PHYS 280 Group Website
The Greene group studies a wide variety of physical phenomena using primarily methods from theoretical atomic, molecular, and optical physics. The phenomena treated range from low energy few-body processes to many-body atomic, molecular and condensed matter systems using a diverse set of theoretical techniques. In the few-body regime, our group examines the behavior of neutral atoms under the influence of radio frequency light or synthetic gauge fields, in addition to conventional electron or photon collision phenomena from low energies up to the X-ray regime. This area also includes studies of highly excited Rydberg atoms interacting with external fields or other neutral atoms. Additional explorations in the few--body regime tackle fundamentally challenging problems involving cold chemistry and the photoassociation of atoms and ions. A recent direction is an extension of our few-body techniques into the realm of many-body physics in a treatment of the two-dimensional quantum Hall system. While the variety of topics considered is far-ranging, all of these studies are connected by the fact that these are quantum mechanical systems with nonperturbative interactions that are highly challenging for existing theoretical methods.
-
Vladimir Shalaev - Co-Chair of Internal Faculty Advisory Board
Robert and Anne Burnett Distinguished Professor of Electrical and Computer Engineering
BRK 1027A Group Website
Quantum Nanophotonics studies light-matter interaction in systems with typical size below the diffraction limit. Examples of such systems include nanoscale plasmonic resonators, waveguides, metamaterials and metasurfaces coupled to single-photon emitters such as color centers or quantum dots. In these systems, the interaction between photons and emitters can be much stronger than in a homogenous environment, leading to a number of phenomena such as spontaneous lifetime reduction and coherent quantum dynamics that could lead to controlled entanglement of several emitters. The richness of near-field phenomena allows to further expand the engineering of light-matter interaction, by achieving high plasmon emission directionality and control of polarization. In addition, because the increase in interaction is due to small mode volumes rather than strong resonances, these effects can be rather broadband and apply to a great variety of emitters. Driven by the applications of quantum control of light and matter, our group is developing efficient room-temperature compact on-chip configurations of quantum systems coupled to nanophotonic structures. Building on our expertise in the field of metamaterials and nanophotonics, we conceive new tunable CMOS-compatible materials and practical designs that will lead to a new generation of single-photon sources, quantum registers, detectors and quantum frequency converters for quantum communication, quantum computation and sensing.
Faculty
-
Hany Abdel-Khalik
Professor of Nuclear Engineering
FLEX 1041B Group Website
The CYbersecurity & data aNalytics for Industrial Control Systems (CYNICS) group led by Dr. Hany Abdel-Khalik develops novel solutions to engineering problems using physics-based and data-driven methodologies with a focus on uncertainty quantification (UQ) and model validation, reduced order modeling (ROM), cybersecurity, and artificial intelligence (AI) applications.
-
Vaneet Aggarwal
Associate Professor of Industrial Engineering, Associate Professor of Electrical and Computer Engineering (by courtesy)
GRIS 260 Group Website
Networking and Communications, Machine Learning, Cloud Computing, Smart Grids, Information and Coding Theory
-
Hadiseh Alaeian
Assistant Professor of Electrical and Computer Engineering, Assistant Professor of Physics and Astronomy
BRK 1291 Group Website
My research focuses on hybrid, scalable, and integrated photonic quantum technologies. In particular, I am interested in theoretical and experimental investigations of interacting and correlated open quantum optical systems. We engineer light-matter interactions and employ highly excited Rydberg states to create large optical non-linearity, which leads to exotic states of light required for many different quantum technologies based on photons.
-
Arnab Banerjee
Assistant Professor of Physics and Astronomy
PHYS 168
A significant amount of human progress has been driven by the discovery and control materials with exceptional properties. This includes the development of modern computers which derived from the control of Silicon or the electric cars which have followed the advancement of Lithium batteries. In the past few decades, the power of material-derived electronics has grown exponentially fast as described by Moore's Law. However, it is now very apparent that the growth will slow down unless we can capture and control the quantum effects in materials that arise from quantum zero-point motion and Heisenberg's uncertainty. How do we do that? The goal of my research is to understand the quantum properties of materials towards making them useful for tomorrow's technology. My research provides insights into new quantum phenomenology in new magnetic materials both in the bulk and device geometries using reciprocal space, such as neutron scattering, and millikelvin transport techniques when the data is mapped into appropriate, preferably quantum models to capture the effects of zero-point motion, fractionalization, quantization, and coherence.
-
Peter Bermel
Professor of Electrical and Computer Engineering
BRK 2270 Group Website
Prof. Bermel's research focuses on improving the performance of photovoltaic, thermophotovoltaic, and nonlinear systems using the principles of nanophotonics. Key enabling techniques for his work include electromagnetic and electronic theory, modeling, simulation, fabrication, and characterization.
-
David Bernal Neira
Assistant Professor of Chemical Engineering
We work on optimization problems with applications in science and engineering and have experience in the study, design, and implementation of such algorithms using hybrid and novel hardware technologies, including quantum computing, graphical processing units, and classical computing. Our research focus on applications related to challenging problems in chemical, process, and energy systems engineering.
-
Sunil Bhave
Professor of Electrical and Computer Engineering
BRK 2021 Group Website
In the OxideMEMS Lab we explore inter-domain coupling in Opto-mechanical, Spin-Acoustic and Atom-MEMS devices. We strive to leverage our understanding of these coupled systems to design and fabricate inertial sensors, clocks, frequency combs and computing and microwave sub-systems.
-
Rudro Biswas
Assistant Professor of Physics and Astronomy
PHYS 54 Group Website
Theoretical Condensed Matter
-
Alexandra Boltasseva
Ron And Dotty Garvin Tonjes Distinguished Professor of Electrical and Computer Engineering
BRK 1295 Group Website
Alternative Plasmonic Materials, Plasmonics with Graphene and Beyond, Integrated Nanophotonic Devices with Alternative Materials, Optical Properties of Alternative Plasmonic Materials, Transparent Conductive Oxides (TCOs) for Metasurface and Epsilon-Near-Zero (ENZ) Applications, Novel Plasmo-Fluidics, Glancing Angle Deposition, Lasing with 3-D Plasmonic Nanorod Metamaterials
-
Erica Carlson
150th Anniversary Professor of Physics and Astronomy
PHYS 262 Group Website
Our research group focuses on condensed matter theory, studying the electronic properties of novel materials. Current group interests include high temperature superconductivity, strongly correlated electrons, liquid crystalline phases of electrons, and soft electronic matter.
-
Yong Chen - Director of Purdue Quantum Science and Engineering Institute
Karl Lark-Horovitz Professor of Physics and Astronomy, Professor of Electrical and Computer Engineering
BRK 1287B Group Website
The Quantum Matter & Devices (QMD) Lab exploits quantum physics to manipulate electrons, photons and atoms in quantum materials and artificial quantum systems, with the aim to uncover novel quantum phenomena & new states of matter and to explore innovative applications in quantum information processing or nanotechnology (such as nanoelectronics and nanosensors). Our research topics include both nano/solid state physics (graphene & 2D materials, topological insulators), atomic/molecular physics (Bose-Einstein condensates, polar molecules) and related applications.
-
Weng Chew
Distinguished Professor of Electrical and Computer Engineering
WANG 3053 Group Website
Electromagnetics, fast and efficient computational algorithms for solving electromagnetic scattering and multiphysics problems
-
Gabor Csathy
Professor of Physics And Astronomy
PHYS 56
New Physics in 2D Electrons (new phases in the low density and low temperature regime), BCS-like Pairing of Composite Fermions, Non-Abelian Statistics and Possible Applications for Quantum Computing, Solid Phases in Electronic Systems, Spin Physics in Low Dimensional Semiconductors
-
Xingshan Cui
Associate Professor of Mathematics
MATH 646 Group Website
Topological quantum field theory, higher category theory, low dimensional topology, quantum invariants, topological quantum computation, quantum information.
-
Supriyo Datta
Thomas Duncan Distinguished Professor of Electrical and Computer Engineering
WANG 3047 Group Website
Spin electronics, nanoscale energy conversion, molecular electronics and mesoscopic superconductivity
-
Stephen Durbin
Professor of Physics and Astronomy
PHYS 166, PHYS 175 Group Website
Ultrafast x-ray dynamics in semiconductors, X-ray physics at synchrotrons, X-ray free electron laser
-
Daniel Elliott
Professor of Electrical and Computer Engineering, Professor of Physics and Astronomy
MSEE 258 Group Website
In our laboratory, we examine fundamental questions of interactions between coherent light and atoms or small molecules. We measure extremely small interaction strengths, and we create and control extremely cold dipole molecules.
-
Christopher Greene - Co-Chair of Internal Faculty Advisory Board
Albert Overhauser Distinguished Professor of Physics and Astronomy
PHYS 280 Group Website
The Greene group studies a wide variety of physical phenomena using primarily methods from theoretical atomic, molecular, and optical physics. The phenomena treated range from low energy few-body processes to many-body atomic, molecular and condensed matter systems using a diverse set of theoretical techniques. In the few-body regime, our group examines the behavior of neutral atoms under the influence of radio frequency light or synthetic gauge fields, in addition to conventional electron or photon collision phenomena from low energies up to the X-ray regime. This area also includes studies of highly excited Rydberg atoms interacting with external fields or other neutral atoms. Additional explorations in the few--body regime tackle fundamentally challenging problems involving cold chemistry and the photoassociation of atoms and ions. A recent direction is an extension of our few-body techniques into the realm of many-body physics in a treatment of the two-dimensional quantum Hall system. While the variety of topics considered is far-ranging, all of these studies are connected by the fact that these are quantum mechanical systems with nonperturbative interactions that are highly challenging for existing theoretical methods.
-
Jonathan Hood
Assistant Professor of Chemistry, Assistant Professor of Physics and Astronomy
BRWN B155 Group Website
The goal of our experiment is to trap single ultracold molecules in optical tweezers with complete control of their internal and motional quantum states. Ultracold dipolar molecules offer a number of exciting new prospects for quantum technologies and for studying and controlling quantum chemistry. In our lab, we will assemble ultracold molecules from individual atoms using optical tweezers. The experiment starts by loading exactly two atoms into separate optical tweezers and then cooling them to the lowest possible motional state: the ground state of the tweezer harmonic oscillator. The two atoms are then adiabatically merged together, associated into a loosely-bound molecule, and then coherently transferred to a single deeply bound vibrational, rotational, and hyperfine state without motional heating. The optical tweezer array of molecules can then be reconfigured to for example bring two molecules together for a quantum gate, or to bring two molecules together for a reaction, or even to create a fully filled lattice of molecules to simulate a quantum spin model. Quantum Computation and Simulation - A dipolar molecule has a permanent dipole resulting in long-range interactions between molecules - much stronger than the magnetic dipole interactions of neutral atoms. The long-lived ground rotational states of ultracold molecules are exciting new candidates for qubits in quantum computation or in quantum simulations. Ultracold Quantum Chemistry - At ultracold temperatures, the de Broglie wavelength of a molecule is large compared to the interaction lengths scales, and quantum phenomena can dramatically change the interactions and reaction dynamics. For example, if two molecules are identical fermions, then the reaction can be suppressed due to the Pauli exclusion principle. Reaction dynamics can be dominated by quantum tunneling, or can be enhanced through Feshbach resonances with bound states. In addition, we see to control the reactions, effectively turning them on or off, by applying electric or magnetic fields, which orient the dipoles and greatly modifies the long-range potential.
-
Mahdi Hosseini
Associate Professor of Electrical and Computer Engineering
BRK 2274 Group Website
Strong and coherent interaction of photons with matter is the corner stone of the future quantum optical technologies. Engineering coherent and efficient light-matter interactions at the single photon level using chip-scale devices is the grand challenge of the quantum photonic technology. Interaction of that kind enables new and exciting applications including secure optical communication, photonic quantum computing and quantum sensing. In our group we are interested in studying strong quantum interaction of light with real and artificial atoms and its role in quantum optical communication, computation and sensing. We work towards developing a hybrid and scalable photonic network that operates at the telecommunication wavelength.
-
Libai Huang
Professor of Chemistry
BRWN B135B Group Website
Spatial and temporal imaging of energy and charge transport in solar energy conversion systems - We employ ultrafast spectroscopy combined with optical microscopy and scanning probe microscopy to achieve simultaneous ultrafast time resolution and nanometer spatial resolution. This research program aims at providing spatial maps of carrier dynamics, and allowing for imaging energy and charge propagation in space directly. This is an exciting new frontier that combines advanced imaging and dynamics techniques to address fundamental questions regarding energy flow in individual nanostructures, organic materials, solar cells, and photosynthetic systems. Direct imaging of exciton and charge transport in solar cell active layers - We have recently developed ultrafast microscopy as a novel means to directly visualize exciton and charge transport with ~ 200 fs temporal resolution and ~ 50 nm spatial resolution in perovskite and organic solar cells. Recently, our group has visualized charge transport directly in perovskite solar cells. With innovative approaches that combine spatial and temporal resolutions, we revealed a new singlet-mediated triplet transport mechanism in certain singlet fission materials, which leads to favorable long-range triplet exciton diffusion for solar cell applications. Probing charge and exciton dynamics at the individual nanostructure level - Our group has pioneered the use of optical pump-probe techniques for imaging ultrafast charge and exciton dynamics at the individual nanostructure level to resolve energy relaxation pathways that are not accessible by traditional ensemble methods. We have spatially resolved carrier dynamics in graphene, individual single-walled carbon nanotubes, and two-dimensional semiconductors such as MoS2 to elucidate the role of the environment in modulating relaxation pathways.
-
Chen-Lung Hung
Associate Professor Physics and Astronomy
PHYS 266 Group Website
We are interested in using tabletop atomic, molecular and optical (AMO) systems to engineer novel quantum materials and study intriguing phenomena discussed across disciplines, from condensed matter to cosmology. To address topics over such a wide range, we exploit physics governed by universality, with which a cold cloud of dilute gases, for example, can behave similarly as an exotic quantum material in solid states, or even as a cosmic fluid in the early universe. AMO systems allow us to apply quantum control and measurements with great precision, offering a clean designer platform to explore fundamental issues in quantum mechanics, field theory, and statistical physics.
-
Zubin Jacob
Professor of Electrical and Computer Engineering
BRK 2293 Group Website
Our group’s research focusses broadly at the interface of quantum photonics, quantum materials and nanotechnology. We are involved in both theory and experiment while simultaneously collaborating with a broad range of faculty at Purdue and around the world. One recurring theme in our research is the study of thermal and quantum noise inside materials and their consequences on dipole-dipole interactions, vacuum forces and single photons.
-
Andreas Jung
Associate Professor of Physics and Astronomy
PHYS 374 Group Website
The research focus of the group is on understanding the structure of the most fundamental building blocks of matter. The instruments required to shed more light on the origin of the structure of elementary particles and their masses are huge. Members of the group perform measurements using data collected with the Compact Muon Solenoid (CMS) experiment at the Large Hadron Collider (CERN). Enormous amounts of data are needed to find out more about this most fundamental question. Amongst all elementary particles the top quark is the most special quark with a mass close to that of an entire gold atom. Our current understanding is that the Higgs mechanism gives mass to the top quark. The measurements of the group are employing data enriched in top quarks (and also Higgs bosons) to study very precisely the special connection between those two fundamental particles and to search for any evidence of contributions beyond the Standard Model. We are also involved in Research and Development for novel silicon pixel detectors and low mass support structures. This research involves cutting edge materials such as carbon fiber, carbon foam and light-weight Aluminum alloys for example Aluminum Carbon fiber. The in-house Purdue Silicon Detector Lab, or PSDL, allows to produce and build support structure prototypes and silicon detector modules. We perform thermal simulations and comparisons to data taken with a CO2 cooling setup, and we collaborate for this research with Cornell, Fermilab, CERN, and other institutes in the US, Europe and world-wide.
-
Sabre Kais
Distinguished Professor of Chemistry, Distinguished Professor of Electrical and Computer Engineering
WTHR 265G Group Website
Electronic Structure of Finite Systems (Finite Size Scaling Method in Quantum Mechanics, Dimensional Scaling and Critical Phenomena, Renormalization Group Methods for Electronic Structure, Pivot Method for Global Optimization), Quantum Information and Quantum Computing (Adiabatic Quantum Computing, Study of Entanglement and Quantum Phase Transitions, Quantum Computing and Quantum Algorithms, Overview of Kais Research in Quantum Information and Computation)
-
Birgit Kaufmann
Professor of Mathematics, Professor of Physics and Astronomy
MATH 748 Group Website
Mathematical Physics Non-equilibrium systems Quantum wire networks from triply-periodic minimal surfaces Finite-size scaling in atomic models
-
Ralph Kaufmann
Professor of Mathematics
MATH 722 Group Website
Algebraic Topology, Algebraic Geometry, Mathematical Physics, Higher Stuctures. Feynman Categories String Topology and Related Operations such as Deligne Conjectures Hopf Algebras Quantum Cohomology and Mirror Symmetry Stringy Phenomena for Stacks/Orbifolds Geometry of Moduli and Teichmueller Spaces Noncommutative Geometry Nano Wire Networks and Topological Insulators 2+1 dim TQFT and its Extensions
-
Alexander Kildishev
Professor of Electrical and Computer Engineering
BRK 1264 Group Website
Dr. Kildishev?s research directions in the area of fields and optics (FO) include computational nanophotonics and nanoscale multiphysics, metasurfaces and metamaterials, 2D and refractory plasmonics, transformation optics, nanolasers and spasers, thermophotovoltaics, and super-resolution imaging techniques and devices.
-
Young Kim
Professor of Biomedical Engineering
MJIS 3027 Group Website
1. Metamaterials in nature and Anderson localization in biological media 2. Quantum-based physically unclonable functions 3. Virtual hyperspectral imaging (VHI) and global bioengineering 4. Photocatalysis using biomaterials and bioenergy conversion 5. Fundamental understanding of light-tissue interactions 6. Bridging bioengineering and epidemiology/biostatistics
-
Gerhard Klimeck
Elmore Professor of Electrical and Computer Engineering
DLR 103 Group Website
The Nanoelectronic Modeling Group works in the area of nanoelectronics where we try to better the understanding of electron flow through nano-scale devices. The effort on modeling and simulation is heavily computer based. We try to connect to experimental results which we try to explain or even predict experiments.
-
Martin Kruczenski
Professor of Physics and Astronomy
PHYS 252 Group Website
String Theory and its connections to Gauge Theory (How strings emerge from gauge theory in the large N limit. Applications to QCD; String descriptions of confining gauge theories), String Theory and Blackhole Physics (Supergravity)
-
Tillmann Kubis
Katherine Ngai Pesic & Silvaco Associate Professor of Electrical and Computer Engineering
FLEX 3041A Group Website
The Kubis group applies quantum transport methods on a variety of many-particle problems – ranging from properties of irregular or imperfect materials and interfaces, novel nanotransistors and chemical reactions of molecules in solution and at interfaces. Most of their methods get implemented into software that runs on supercomputers and is frequently used in industry.
-
Rafael Lang
Professor of Physics and Astronomy
PHYS 313 Group Website
What is the Universe made of? All we know today is that we don't know what most of the stuff in the Universe really is. We have names for it, calling it Dark Matter and Dark Energy, but we don't know its true Nature. Is Dark Matter made of a new particle species? And if so, what are its properties? How is it distributed? Can Dark Matter interact with us? I build and run detectors that try to shed light on these issues, in particular utilizing liquid noble gases. I am a member of the ???XENON collaboration that use a liquid xenon target to search for rare Dark Matter interactions. I am in particular interested in unconventional signatures of Dark Matter. Further, I am working on using these detectors to detect neutrinos from processes in our Sun or from supernova explosions across the Milky Way. In addition to these collaborative efforts, I operate dedicated R&D setups at my lab at Purdue to advance these detector technologies further.
-
Nima Lashkari
Assistant Professor of Physics and Astronomy
PHYS 253 Group Website
The current focus of my research is the study of non-perturbative phenomena in quantum field theory and gravity through the lens of quantum information theory.
-
Tongcang Li
Professor of Physics and Astronomy, Professor of Electrical and Computer Engineering
PHYS 52 Group Website
Recently developments in quantum optomechanics provide new opportunities to study macroscopic quantum mechanics and create ultrasensitive detectors. Creating large quantum superposition states (Schr?dinger’s cat) with massive objects is one of the most challenging goals in macroscopic quantum mechanics. We have optically levitated nanodiamonds with nitrogen-vacancy (NV) centers in vacuum. We plan to generate large spatial superposition states and arbitrary phonon Fock states of levitated nanodiamonds using the NV spin-optomechanical coupling with the assistance of a strong magnetic field gradient. The large spatial superposition states can be used to study objective collapse theories of quantum mechanics. A levitated nano-optomechancial system will also be a sensitive force detector. We are also interested in studying novel macroscopic quantum behaviors. Recently, we proposed a straightforward method to create quantum superposition states of a living microorganism by putting a small cryopreserved bacterium on top of an electromechanical oscillator. The internal states of a microorganism, such as the electron spin of a glycine radical, can also be entangled with its center-of-mass motion and teleported to a remote microorganism.
-
Qi-Yu (Grace) Liang
Assistant Professor of Physics and Astronomy
PHYS 264 Group Website
I am an experimental AMO physicist, specializing in quantum optics and degenerate quantum gases. I endeavor to explore interactions of new types, on new platforms and in different parameter regimes to advance our understanding of fundamental physics and open up new research areas. My future research will be a continuation of my current and past work, with enhanced level of control. For example, single-site resolution will allow synthetic gauge fields to be applied locally; confining the atoms tightly in 3D optical lattices will enhance the role of interactions between atoms, permitting the study of the interplay between interactions and spin-orbit coupling; subwavelength-spaced arrays of atoms emerge to be a new interface of light-matter interaction, whose properties could be altered by Rydberg excitations; studying Rydberg composites in platforms with precise control of atoms could uncover interesting new states; and so on.
-
Jing Liu
Associate Professor of Physics and Astronomy
PHYS 62 Group Website
My research focuses on the spatiotemporal dynamics of the DNA and RNA that are the building blocks of life. Understanding these fundamental physical properties will facilitate the diagnosis, treatment, and prevention of cancers, diabetes, and Alzheimer???s Disease. My further research will integrate quantum optics techniques that would enable further advances in these fields.
-
Yuli Lyanda-Geller
Professor of Physics and Astronomy
PHYS 278, BRK 1293 Group Website
Mesoscopic physics and interference phenomena Transport and Optical phenomena in nanostructures Physics of Quantum Information
-
Alex Ruichao Ma
Assistant Professor of Physics and Astronomy
PHYS 252 Group Website
Quantum many-body physics and quantum information science in superconducting circuits. We will be developing new methods to create and study synthetic quantum materials made of interacting microwave photons in superconducting circuits. Taking advantage of the coherent control and flexibility of the superconducting circuit platform, we will build “analog quantum simulators” to gain microscopic understanding of novel material properties arising from the competition between quantum fluctuation, interactions and topology; and investigate many-body dynamics in non-equilibrium and open quantum systems. Our research will be at the intersection of condensed matter, AMO physics, and quantum information science. We will also be exploring potentials of building more versatile analog quantum simulators by integrating superconducting circuits with other solid state or AMO systems.
-
Michael Manfra
Bill and Dee O’Brien Distinguished Professor of Physics and Astronomy, Professor of Materials Engineering, Professor of Electrical and Computer Engineering, Scientific Director, Microsoft Quantum Lab West Lafayette
PHYS 84 Group Website
The Quantum Semiconductor Systems group studies the behavior of electrons confined in reduced dimensional systems subject to strong mutual interactions. Researchers in the Quantum Semiconductor Systems group use a variety of techniques including semiconductor growth by molecular beam epitaxy (MBE), nanofabrication and low-temperature transport to explore this exciting field. MBE is a process to fabricate crystalline semiconductor heterostructures for the study of novel physical properties and solid-state device technology. In an MBE system we can grow heterostructures of dissimilar materials with atomic monolayer resolution. This allows us to explore the properties of strongly interacting electrons in two dimensions. We also exploit the nanofabrication facilities at the Birck Nanotechnology Center to further confine electrons in 1D (quantum wires) and 0D (quantum dots) structures. While these reduced dimensional systems exhibit many emergent phenomena due to collective behavior, they also hold promise as platforms for quantum computing. The Quantum Semiconductor Systems group uses electrical transport experiments at temperatures down to T=10mK and magnetic fields up to 15Tesla to interrogate the samples we create. We also use MBE to pursue novel light-emitting sources in the Al(In)GaN heterostructure system.
-
Saeed Mohammadi
Professor of Electrical and Computer Engineering
BRK 2264 Group Website
VLSI and Circuits, Microelectronics and Nanotechnology
-
Evgenii Narimanov
Elmore Professor of Electrical and Computer Engineering
BRK 1293
Negative Index (Meta) materials, Optical systems with ray-chaotic dynamics, Information-theoretical description of nonlinear fiber-optical systems, Non-linear dynamics
-
Laimei Nie
Assistant Professor of Physics and Astronomy
PHYS 248
I am broadly interested in the quantum mechanical properties of systems with many particles, such as crystals and ultracold atomic gases. Interesting behaviors tend to occur in both equilibrium and non-equilibrium states of the system when the particles are strongly interacting with each other, and/or when there is disorder (impurities). Previously I have worked on the equilibrium aspects, and currently my research is focused on characterizing non-equilibrium physics (dynamics) in such systems. Examples include chaotic dynamics, where initial information spreads very fast under the time evolution, and localization, where the degrees of freedom are frozen due to the interplay of interactions and disorder. Decoding these fascinating non-equilibrium phenomena will assist us in understanding and designing quantum materials and synthetic quantum matter. Furthermore, it is also connected to some of the most exciting recent developments in high energy physics and quantum information, including resolving the black hole information paradox and building quantum computers.
-
Minghao Qi
Professor of Electrical and Computer Engineering
BRK 1297
Nanotechnology, especially 3D nanofabrication and low-cost nanolithography; micro and nanophotonics, with emphasis on 3D photonic crystals and integrated Si photonic circuits; thermophotovoltaics and solar cells
-
Francis Robicheaux
Professor of Physics and Astronomy
PHYS 284 Group Website
My research area is Theoretical Atomic Physics, mainly focusing on time dependent atomic phenomena, highly excited (Rydberg) atoms, electron scattering, strong fields and ultracold plasmas. My research group typically consists of undergrads, grad students and postdocs. I'm a member of the ALPHA collaboration which was the first group to trap the antimatter version of the Hydrogen atom and the first group to quantitatively measure some of its properties.
-
Leonid Rokhinson
Professor of Physics and Astronomy
PHYS 60 Group Website
Electron transport in mesoscopic systems Spintronics and spin interactions Magnetic materials and devices Quantum information processing Topologically non-trivial states of matter Nanofabrication Novel materials and devices
-
Thomas Roth
Assistant Professor of Electrical and Computer Engineering
WANG 3063 Group Website
The Computational Quantum Electromagnetics Lab is interested in the development of robust and efficient multiscale and multiphysics modeling methods to help accelerate the design process of quantum electromagnetic technologies. These technologies will help form the hardware layer of many forms of quantum computers, quantum sensors, and quantum communication systems. We approach these problems by developing novel quantum theoretical frameworks that can leverage the latest advances in computational electromagnetics research.
-
Eric Samperton
Assistant Professor of Computer Science, Assistant Professor of Mathematics
MATH MATH 402
Quantum topology and algebra, complexity and algorithms, quantum computing
-
Vladimir Shalaev - Co-Chair of Internal Faculty Advisory Board
Robert and Anne Burnett Distinguished Professor of Electrical and Computer Engineering
BRK 1027A Group Website
Quantum Nanophotonics studies light-matter interaction in systems with typical size below the diffraction limit. Examples of such systems include nanoscale plasmonic resonators, waveguides, metamaterials and metasurfaces coupled to single-photon emitters such as color centers or quantum dots. In these systems, the interaction between photons and emitters can be much stronger than in a homogenous environment, leading to a number of phenomena such as spontaneous lifetime reduction and coherent quantum dynamics that could lead to controlled entanglement of several emitters. The richness of near-field phenomena allows to further expand the engineering of light-matter interaction, by achieving high plasmon emission directionality and control of polarization. In addition, because the increase in interaction is due to small mode volumes rather than strong resonances, these effects can be rather broadband and apply to a great variety of emitters. Driven by the applications of quantum control of light and matter, our group is developing efficient room-temperature compact on-chip configurations of quantum systems coupled to nanophotonic structures. Building on our expertise in the field of metamaterials and nanophotonics, we conceive new tunable CMOS-compatible materials and practical designs that will lead to a new generation of single-photon sources, quantum registers, detectors and quantum frequency converters for quantum communication, quantum computation and sensing.
-
Niranjan Shivaram
Assistant Professor Physics and Astronomy
PHYS 262 Group Website
Research in my group is focused on using coherent extreme-ultraviolet and soft x-ray light generated by the process of high harmonic generation (HHG) to study electron dynamics in atoms, molecules and materials on femtosecond to attosecond time scales. We use the HHG source in combination with wavelength tunable laser pulses from an optical parametric amplifier to obtain time-resolved information using multiple probing schemes. Current focus is on studying ultrafast dynamics near conical intersections in molecules using a new approach based on Optical Kerr-effect Spectroscopy. This will be complemented by photo-electron spectroscopy experiments using a velocity-map imaging spectrometer. In addition to this, we are interested in using X-ray Free Electron Laser sources such as the Linac Coherent Light Source (LCLS) to study ultrafast dynamics in molecular systems.
-
Wojciech Szpankowski
Saul Rosen Distinguished Professor of Computer Science
LWSN 1201 Group Website
Analysis and design of algorithms, multimedia data compression, bioinformatics, information theory, random structures, analytic combinatorics, performance evaluation, networking, stability problems in distributed systems, modeling of computer systems and computer communication networks, queueing theory, and operations research.
-
Pramey Upadhyaya
Assistant Professor of Electrical And Computer Engineering
WANG 3065 Group Website
Magnetism, classical and quantum spintronics, next-generation information processing & bio-devices.
-
Jukka Vayrynen
Assistant Professor of Physics and Astronomy
PHYS 282 Group Website
Our group is interested in studying open problems related to various quantum computing and microelectronics platforms. For example, our research aims to understand limiting factors of qubit coherence in quantum computing devices. In particular we have focused on topological and conventional superconductors in the condensed matter setting.
-
Valentin Walther
Assistant Professor of Chemistry
WTHR 265B Group Website
We develop methods and schemes to observe and characterize the effects of strong quantum correlations and investigate how they could be used in quantum information science. Our group has dual interests in atomic systems and semiconductors. In atomic platforms, we work on new ideas for some of the highest precision quantum devices, such as arrays of neutral Rydberg atoms and novel types of Rydberg molecules (so-called macrodimers). In semiconductors, our goal is to develop a theoretical description of the excitonic Rydberg states, their interactions and how they affect the (quantum) optical properties of the semiconductor.
-
Adam Wasserman
Professor of Chemistry
WTHR 265B Group Website
The Wasserman group works on the development of Density Functional Theory (DFT) for both ground-state and time-dependent quantum chemistry. In particular, Partition Density Functional Theory (PDFT) is a formally exact method for calculating the energy and density of electronic systems via self-consistent calculations on isolated fragments. PDFT allows for an accurate and efficient solution of the many-electron Schrodinger equation for complex molecules and materials, physically-motivated approximations are needed to calculate the partition potentials that enter the theory. Much of our research effort goes into developing and testing such approximations.
-
Andrew Weiner
Scifres Family Distinguished Professor of Electrical and Computer Engineering
BRK 1295 Group Website
Entangled photons exhibit correlations that are unattainable with classical light. Their stronger-than-classical correlations make them desirable in applications ranging from secure communications to high-speed computation. Although, many degrees of freedom for entanglement exist, our research focuses on developing novel techniques for controlling time-frequency entangled photons (“biphotons”) that would be applicable in quantum communication. Most of our manipulation schemes have utilized broadband biphotons generated from spontaneous parametric down-conversion in periodically poled lithium niobate waveguides. However, we are also now exploring photon pair generation using silicon nitride microring resonators, a platform that could lead us to on-chip time-frequency manipulations.
-
Peide Ye
Richard J. And Mary Jo Schwartz Professor of Electrical and Computer Engineering
BRK 1291 Group Website
Semiconductor physics and devices, Nano-structures and nano-fabrications, Quantum/spin-transport, Atomic layer deposition, High-k/III-V and Ge device integration, High-performance III-V and Ge MOSFETs, High-k/2D integration, High-performance 2D devices, 2D spintronics, All oxide electronics, and wide bandgap semiconductor GaN and Ga2O3 power electronics.
-
Qi Zhou
Professor of Physics and Astronomy
PHYS 268 Group Website
We study a wide range of topics in quantum gases and related fields, such as synthetic gauge fields for ultracold atoms, strongly interacting bosons and fermions, quantum many-body dynamics, and connections between few-body and many-body physics, among many others.
Projects
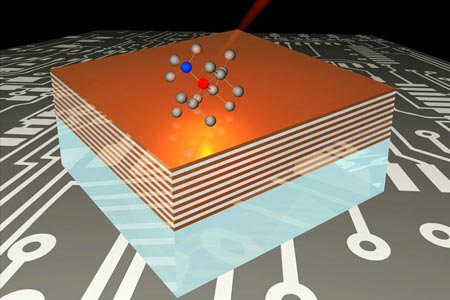
Quantum nanophotonics with color centers in diamond »
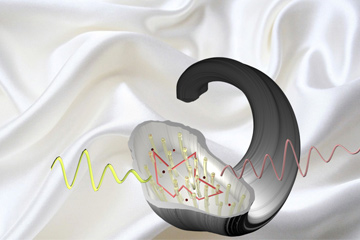
Scalable Wearable Systems for Plasmonics and Photocatalysis »
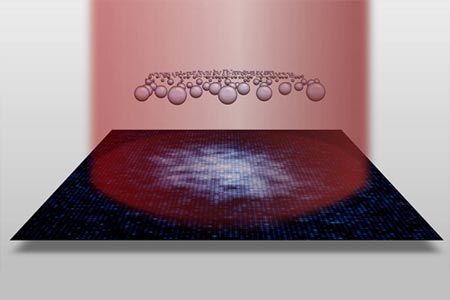
Exploring many-body physics with strong atom-light interactions »
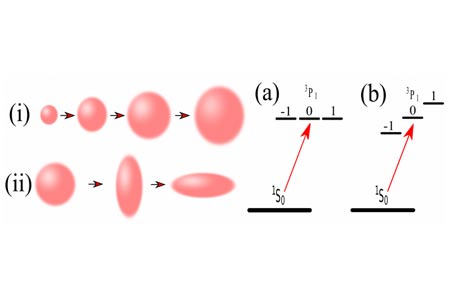
Dipole-dipole interaction between atoms »
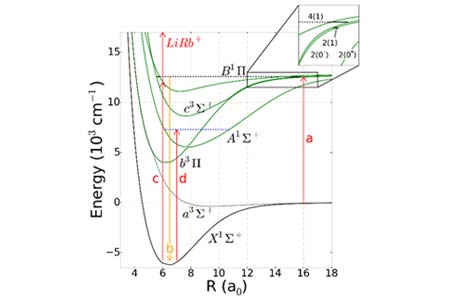
Ultra-cold LiRb molecules »
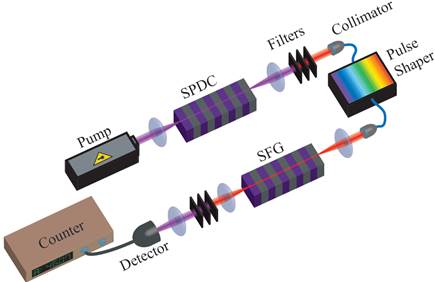
Shaping of Entangled Photons »